Experimental infection of a porcine kidney cell line with hepatitis A virus
Article information
Abstract
The hepatitis A virus (HAV) induces severe acute liver injury and is adapted to human and monkey cell lines but not other cells. In this study, the HAV was inoculated into porcine kidney (PK-15) cells to determine its infectivity in porcine cells. The growth pattern of the HAV in PK-15 cells was compared with its growth pattern in fetal rhesus kidney (FRhK-4) cells. The growth of HAV was less efficient in PK-15 cells. In conclusion, HAV replication was verified in PK-15 cells for the first time. Further investigations will be needed to identify the HAV-restrictive mechanisms in PK-15 cells.
Hepatitis A is a disease caused by an infection with the hepatitis A virus (HAV). The HAV, a positive-sense single-stranded RNA virus with a 7.5 kb genome, belongs to the genus, Hepatovirus, family, Picornaviridae [1]. The HAV was initially classified as a non-enveloped virus, but a recent study reported that this virus could exist in an enveloped form by hijacking the cellular membranes [2]. The HAV is hepatotropic and produces viremia by replicating in liver tissue. After replication, the virus is released into bile, where the detergent action of bile salts removes the envelope, resulting in its eventual excretion in feces [3]. The virus can cause mild to severe symptoms, including jaundice, fatigue, and abdominal pain, affecting an estimated 1.4 million people yearly. In addition, approximately 113 million people become infected with the HAV but do not develop symptoms [4]. Although the disease is rarely fatal, it can cause debilitating symptoms. In rare cases, however, it can lead to deadly acute liver failure (fulminant hepatitis). Hepatitis A occurs sporadically and in epidemics worldwide and tends to recur cyclically. The HAV is typically transmitted through direct contact with infected individuals or by ingesting contaminated food or water, making it one of the most common causes of foodborne infections. The virus spreads through the fecal-oral route, meaning that it is present in the feces of infected individuals and can be transmitted when contaminated fecal matter enters the mouth [5–7]. Despite the availability of effective vaccines, HAV infections remain a serious public health concern worldwide.
The Human HAV has been propagated successfully in a monkey kidney cell line [8]. Previous studies reported that the HAV only infects humans and non-human primates as its natural hosts [9]. The infectivity of the HAV has also mainly been studied in cell lines originating from humans and non-human primates [10,11]. In contrast, one study suggested that the HAV can infect a porcine kidney cell line, which has not been confirmed in further studies [12]. Interestingly, a previous study observed anti-HAV antibodies in swine sera, and pigs were infected experimentally with the HAV [13]. Therefore, this study was performed to verify the HAV infectivity and replication in the porcine kidney cell line, which is used widely for the pathogenic studies of several viruses.
HAV strain HM-175/18f, clone B (VR-1402), was obtained from the American Type Culture Collection (ATCC, USA). Fetal rhesus kidney (FRhK-4, ATCC no. CRL‐1688) and porcine kidney (PK-15, ATCC no. CCL-33) cells were inoculated with 100 genomic-equivalent (GE) copies of the HAV per cell. Both cell types inoculated with the virus were maintained for three days in Dulbecco’s modified Eagle’s medium supplemented with 4% heat-inactivated fetal bovine serum (Gibco, USA) and an antibiotic-antimycotic reagent (Gibco). Supernatants of the inoculated cells and cell lysates were sampled daily for three days. The viral RNA was extracted from the supernatants using a Patho Gene-spin DNA/RNA kit (iNtRON, Korea), and intracellular RNA was extracted from the cell lysates using an RNeasy Mini RNA isolation kit (Qiagen, Germany) according to the manufacturer’s instructions. Table 1 lists the primers and probes used to detect the HAV RNA in this study. Reverse transcription-quantitative polymerase chain reaction (RT-qPCR) for the detection of viral RNA was performed using an AgPath-ID One-Step RT-PCR kit (Applied Biosystems, USA) and a Smart Cycler thermal cycler (Cepheid, USA). The viral GE copy numbers were calculated from a standard curve generated using the plasmid DNA containing the target sequence of HAV (22–108 bp of HM-175 strain, GenBank No. M14707). The viral genomic copy numbers were divided by 108 GAPDH GE copies to calculate the number of viruses per cell. GAPDH mRNA was quantified using a One Step TB Green PrimeScript RT-PCR Kit (Takara, Japan) and a Light Cycler thermal cycler (Roche, Switzerland). The negative-sense HAV RNA was detected using previously described methods [13]. All experiments were repeated three times to obtain reliable results. FRhK-4 and PK-15 cells were grown on six-well plates until they reached 70% confluence for immunofluorescence assays. The following day, the cells were inoculated with 100 GE copies of VR-1402 per cell. The cells were fixed with 4% paraformaldehyde in PBS (pH 7.4), three days post-inoculation (dpi), and were permeabilized with 0.25% Triton X-100 (Sigma, USA). An anti-HAV polyclonal antibody (ab68579; Abcam, UK) diluted 1:1,000 with PBS was reacted with permeabilized cells. The cell nuclei were stained with 4’,6-diamidino-2-phenylindole (DAPI). The red fluorescent spots of HAV in the cells were observed directly by fluorescence microscopy (EVOS FL Imaging System; Life Technologies, USA).
The primary purpose of this study was to determine if HAV can infect porcine-derived PK-15 cells. FRhK-4 cells derived from a non-human primate were used as a positive control for HAV replication. Extracellular and intracellular viral copy numbers were determined using the cell culture supernatants and cell lysates, respectively, at 0, 1, 2, and 3 dpi. Both cell lines produced HAV from 1 dpi. On the other hand, the viral numbers were significantly higher in FRhK-4 cells than in PK-15 cells at 2 and 3 dpi (Fig. 1A). The intracellular and extracellular viral numbers showed similar patterns. FRhK-4 cells contained significantly higher numbers of intracellular viruses than PK-15 cells at 1, 2, and 3 dpi (Fig. 1B). These results suggest that the HAV can replicate in PK-15 cells but less efficiently than in FRhK-4 cells.
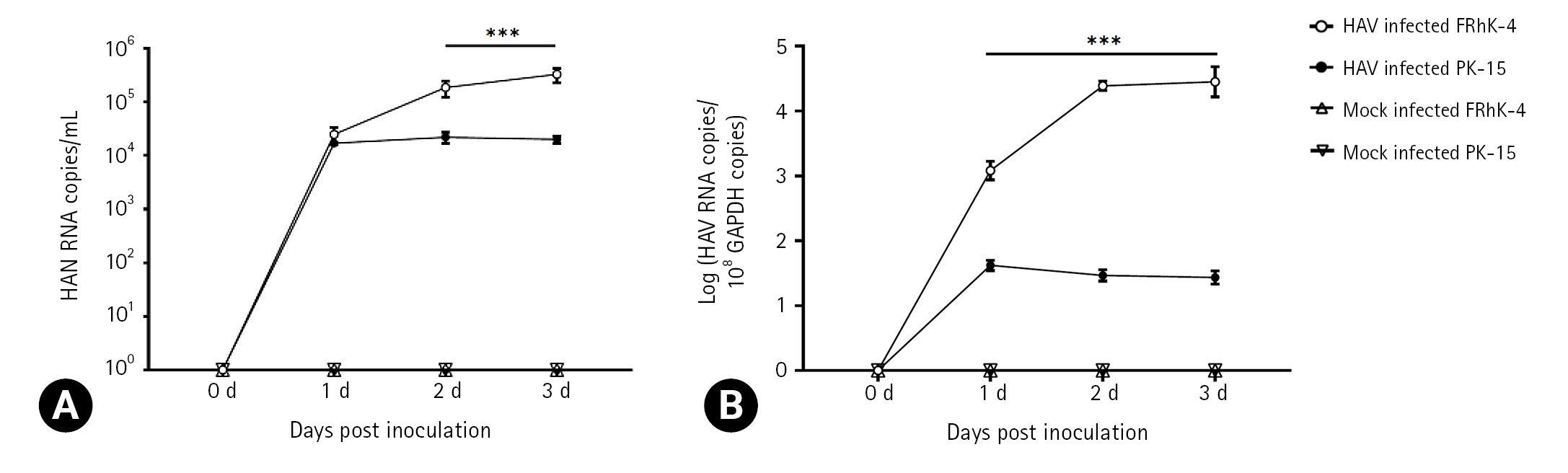
Infection of fetal rhesus kidney (FRhK)-4 cell and porcine kidney (PK)-15 cells with HM-175/18f hepatitis A virus (HAV). The cells were infected with a viral titer of 100 genome equivalents/cell. (A) Extracellular viral RNA copy numbers were determined from the supernatants. (B) Intracellular viral RNA copy numbers were determined from cell lysates by dividing the viral RNA copy number by the GAPDH copy number. In uninfected control cells, HAV was not detected. Bar graphs represent the mean ± standard deviation (n = 3). Data were analyzed by Student t-test. ***p < 0.001.
During replication, the HAV produces a negative-sense intermediate viral RNA, which acts as a template for synthesizing a positive-sense viral RNA [14,15]. The production of a negative-sense viral RNA was determined at 0, 6, 12, 24, 36, 48, and 72 hours post-inoculation (hpi). The negative-sense viral RNA could be identified from 6 to 72 hpi in both cell types (Fig. 2). These results suggested that HAV successfully replicated in both cell lines. The intracellular localization of the HAV was identified in both FRhK-4 and PK-15 cells by an immunofluorescence assay (Fig. 3). The fluorescence spots were observed more prominently in the cytoplasm of FRhK-4 cells compared to PK-15 cells, suggesting a higher efficiency of viral protein synthesis in FRhK-4 cells. This observation aligns with the intracellular viral copy number results, suggesting that viral protein synthesis occurred successfully in both cell types but to a lesser extent in PK-15 cells.

Screening of negative-sense hepatitis A virus (HAV) RNA in fetal rhesus kidney (FRhK)-4 cells (lanes 1, 2, 3, 4, 5, 6, and 7) and porcine kidney (PK)-15 cells (lanes 8, 9, 10, 11, 12, 13, and 14). This assay was performed at 0, 6, 12, 24, 36, 48, and 72 hours post-inoculation (lanes 1&8, 2&9, 3&10, 4&11, 5&12, 6&13, and 7&14, respectively). In the uninfected control cells, negative-sense HAV RNA was not detected (data not shown).

Immunofluorescent staining of hepatitis A virus (HAV)-infected cells three days post-inoculation. Red fluorescent spots demonstrate HAV proteins in the cytoplasm of HAV-infected cells. Nuclei were counterstained with DAPI (blue). × 400.
This study confirmed that HAV can infect and replicate in a porcine-derived cell line. This aligns with a previous study, which reported that pigs could be infected experimentally with the HAV [13]. Hence, HAV replication is not restricted to primates. The adaptation of HAV to different cell lines, including guinea pig cells, can occur through mutations in the 5' non-translated region or other regions [16]. A comparison of the mutated regions of PK-15-adapted HAV could identify the specific mutations required for successful adaptation during infection in pig cells. Further investigations will be needed to explore the potential mutations necessary for a HAV infection in porcine cells and elucidate the underlying mechanisms of adaptation. The in vitro results presented in this paper, together with the in vivo results, suggest that pigs may be another suitable animal model for examining HAV infections and their pathogenesis. On the other hand, the growth of HAV in PK-15 cells was less efficient than in FRhK-4 cells, suggesting some restriction mechanisms for the HAV in PK-15 cells. The precise mechanism of these restrictive actions in PK-15 cells is not known. Further studies, including comparative genomic analysis, host-virus interaction studies, transcriptomic and proteomic profiling, characterization of cellular antiviral responses, and viral adaptation experiments, are needed to elucidate the factors contributing to the limited replication of HAV in PK-15 cells.
Notes
The authors declare no conflict of interest.
Acknowledgements
This research was supported by the Korea Institute of Planning and Evaluation for Technology in Food, Agriculture, Forestry (IPET) through Agriculture, Food, and Rural Affairs Convergence Technologies Program for Educating Creative Global Leader Program, funded by Ministry of Agriculture, Food, and Rural Affairs (MAFRA) (grant number: 320005-04-4-SB0a0).